The Region’s ecosystems remain vulnerable to the effects of legacy as well as current and future coastal development, compounded by interactions with other factors like climate change (Section 6.3). The effects of different land uses and activities combine to modify coastal ecosystems, alter connectivity across landscapes, directly remove and replace intertidal and subtidal marine ecosystems, and create chronic changes in the marine environment. The primary pressure from coastal development is poor water quality from agricultural land use. Barriers to flow across the landscape, and modifications to wetlands, increase the risks posed by poor water quality. By comparison, direct pressures from urban, industrial and mining development are currently relatively minor.
The Reef remains vulnerable to legacy, current and future coastal development
Modifying coastal ecosystems for coastal development limits their ability to provide ecosystem functions and services that support the values of the Reef (Section 3.5). Two key impacts from modifications are the removal of natural vegetation, leading to major erosive processes and altered ecosystem functions and connections across landscapes. Coastal development for agriculture reduces the ability of groundcover in intact natural ecosystems to prevent sediment runoff and nutrient runoff from the Catchment. Agricultural activity is the major cause of erosive processes that contribute to land-based runoff (Section 6.5.1). Both historical and active land clearing expose the land to erosive processes. Land throughout the Catchment has been cleared over the past 150 years for agricultural purposes, and agriculture remains the predominant purpose for contemporary land clearing.1740
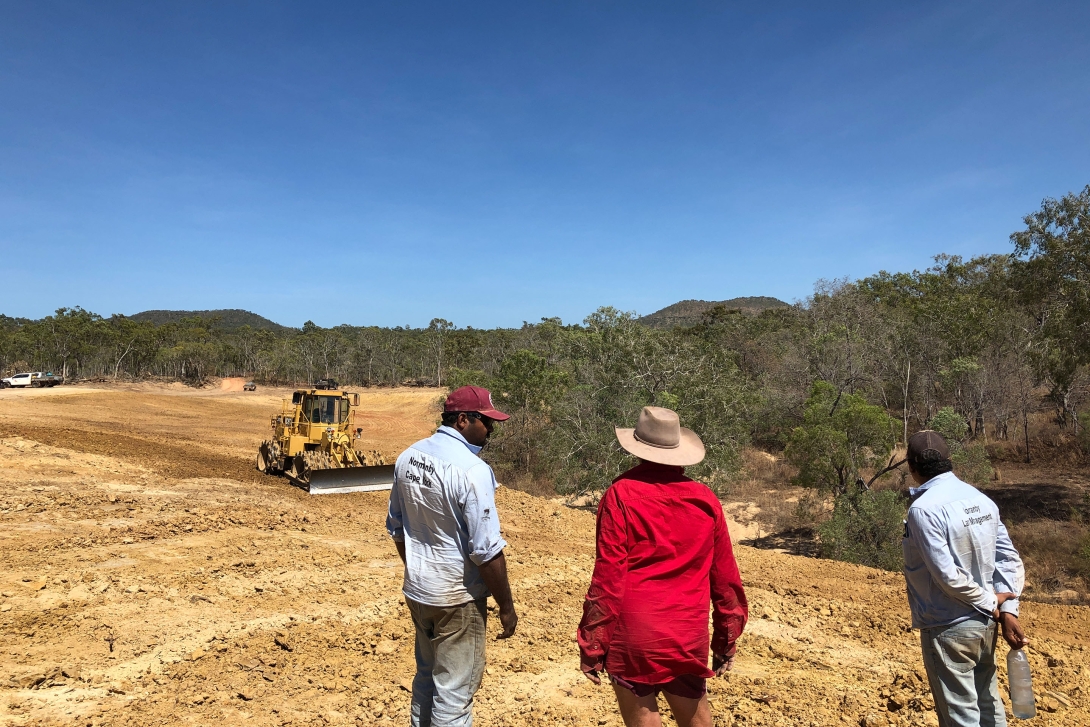
Remediation works in the Catchment are aimed at reducing these sediment and nutrient loads. The extent to which retention or rehabilitation of catchment vegetation can mediate loss of sediments, nutrients and other pollutants is highly variable, depending on the condition and functional traits of vegetation as well as factors like climate and topography.1795 Different forest types do not have equivalent roles in this regard. Channel erosion can be directly linked to the extent of woody riparian vegetation,1796,1770 yet improvements in water quality through streambank rehabilitation are harder to quantify 1797 and require improvements at sufficiently large scales.1798 Streambank rehabilitation work is proceeding in several areas and sediment reductions are assessed using the CSIRO Gully and Streambank Toolbox. For example, the NQ Dry Tropics’ Landholders Driving Change project undertook major gully remediation, streambank and site erosion control works in the Bowen, Broken and Bogie catchments.
Monitored gully remediation sites have shown significant improvements in land condition, vegetation cover and sediment loads.1799,1800 A net increase in private protected areas in the Catchment between 2019 and 2023 (158,415 hectares (1584 square kilometres) of additional area) may also contribute to mitigating the downstream effects of land-based runoff.1801 For example, the conversion of the Springvale Station to a nature refuge, and subsequent destocking, has resulted in significant ground cover benefits in the Normanby catchment. Active erosion control works are underway in many nature refuges across the Catchment.1802 Targeting potential hotspots for erosive losses as priorities for protection can have outsized effects on downstream impacts.1796,1803
Changes to coastal landscapes from clearing and modified hydrology alter ecological functions and the flow of productivity across the Region. The marine ecosystems of the Region rely on land-based ecosystem processes occurring in the landscapes of the Catchment to: supply nutrients and sediments through stream flow and seasonal flooding; slow overland flows;1804 buffer nutrient, sediment and pollution loads through wetland cycling;1805,1806,1807 and deliver animal biomass through food chains, migration and complex life cycles.1808 For example, many tropical snappers, such as mangrove red snapper, spend their juvenile phase in coastal habitats, such as the inland upper tidal reaches of estuaries, while their adult phase is spent on coral reefs. A diversity of crustaceans, fishes, sharks and rays, including many important fisheries species, move between coastal and reef ecosystems to access habitats and food sources supported by land–sea connectivity.1808,1809
This land–sea connectivity is interrupted in landscapes with extensive barriers to flow, degraded wetland condition and increased sediment deposition, which change the extent and distribution of coastal habitats (Section 3.5) and disrupt ecosystem functioning at a variety of scales.1754 While it is well understood that barriers to flow place the lifecycles of many species at risk, relevant species-level ecological information in the Region is still lacking, resulting in an inability to accurately assess and predict the vulnerability of the marine ecosystem to these factors.1808 Long-standing legislative arrangements mean the Region’s exposure to additional impacts from new ponded pastures infrastructure, which create barriers to flow, should be limited.943
The impacts of coastal development manifest over widely varying spatial scales. Broadscale impacts encompass those changes to physical characteristics of the marine environment operating over large areas, whereas local-scale impacts are generally restricted to specific, delimited areas. Localised impacts are generally more amenable to targeted management efforts (Section 7.3.10). Broadscale impacts present unique challenges for management, requiring coordination across jurisdictions and ecosystems.
Broadscale impacts of coastal development
Agriculture, port activities, point source discharge from sewage and aquaculture, and urban and industrial infrastructure have combined to change the regime of physical stressors on the marine ecosystem. Land-based runoff (Section 6.5) presents the greatest affect on vulnerability posed by coastal development. Anthropogenic sediment and nutrient catchment loads affect marine water quality in several ways.1810 Barriers to flow, particularly large dams, can reduce sediment and particulate nutrient runoff. However, fine sediments (particles less than 20 micrometres) are generally poorly trapped in these reservoirs and the overall reduction does not outweigh the effects of other drivers of land-based runoff.959 New large dams can facilitate more intensive land-uses in the Catchment that may contribute to land-based runoff. In-water activities from coastal development also contribute to this altered regime of stressors (for example, marine infrastructure development and impacts of ports Section 5.7.3). Resource extraction and export activities in the state facilitate the global use of fossil fuels, the primary driver of climate change and ocean warming (Section 6.3).
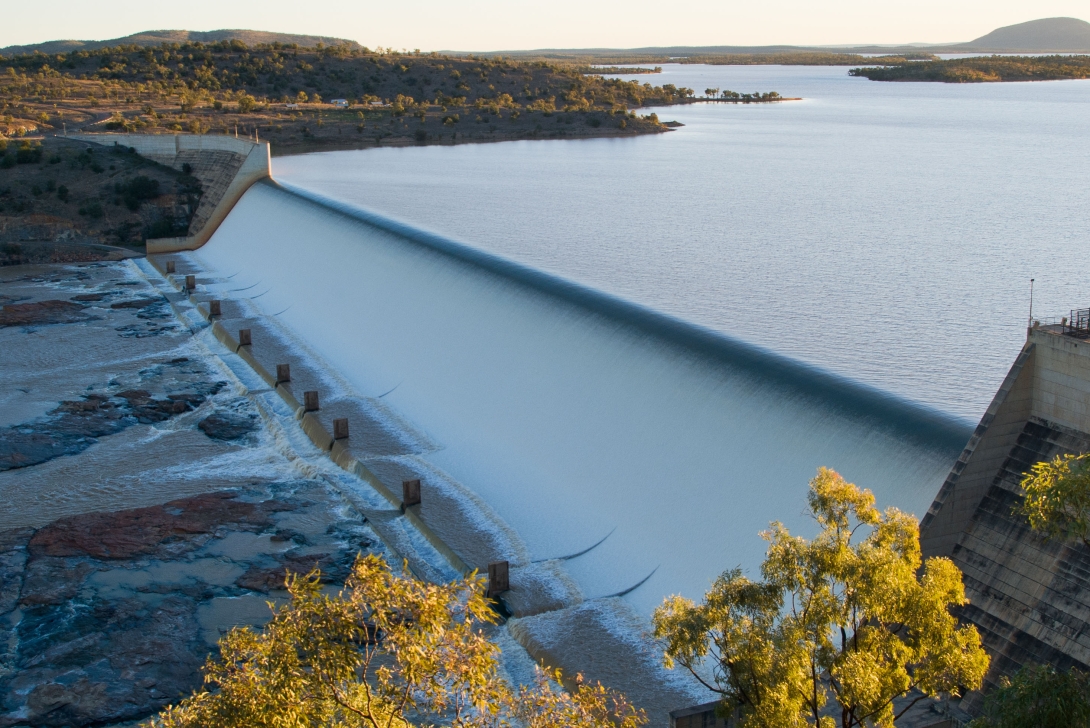
Other chronic changes include artificial light at night from skyglow. Light is a major driving force of key processes in marine ecosystems, and artificial light pollution is known to affect coastal ecosystems by changing schedules of feeding, hiding and resting across trophic levels.1811 Skyglow can inhibit seabird and sea turtle navigation, change foraging and predation patterns, inhibit zooplankton diel vertical migration, and disrupt coral broadcast spawning synchronisation.1812,1813 Marine ecosystems close to the mainland coast are at greatest risk from these chronic changes. Shallow inshore ecosystems, including inshore corals and seagrass, are most vulnerable to the full suite of impacts from coastal development given their proximity to the coast130 and their greater ecological integration with a range of coastal and catchment ecosystems.838
Localised impacts of coastal development
Some activities create unique vulnerabilities that have the potential to cause serious localised damage to the marine ecosystem. Tidal works, such as foreshore seawalls and groynes outside, and encroaching, on the Region, can affect inshore marine habitats by altering local hydrology, permanently removing inshore marine habitats and diminishing local aesthetic attributes. The risk of impacts from these changes is increasing as regional communities expand in the coastal zone.
Direct localised impacts of artificial light adjacent to the Region include disorientation of emerging marine turtle hatchlings as they have a reduced ability to find the sea in the presence of artificial light (Section 2.4.10).
Acid, metals and other contaminants in soils can affect many species at a local scale, both immediately and through accumulation in the food chain. Activities that may disturb acid sulfate soils have been regulated in Queensland since the early 2000s, reducing the risk of exposure of the Region to such impacts.
Mining and other resource activities cover a very small proportion of the Catchment compared to other land uses, but they can expose the Region to impacts that are hard to reverse. Pollutants, such as contaminated tailings water, flowing into the Region or water table are a current and future threat. However, these threats are regulated through existing environmental protection legislation.1814,1815