Changes to the global climate interact with existing climate drivers (Box 6.1) to determine climate patterns in the Region.1538 Changes brought about by global warming can either amplify or reduce the effects of these regional drivers, leading to altered weather patterns and changes in the timing, duration and intensity of extreme events.1539,1540 There is also evidence some drivers are themselves being altered as the climate changes, affecting the way regional climates are linked to distant climate phenomena and causing permanent shifts to drier or wetter conditions in some areas.540,569,1540 For example, recent increases in both the strength and frequency of El Niño–Southern Oscillation events have been observed over various timescales, though it is not yet clear whether these are outside the range of historical variability.1540,1541,1542 It is also projected that future El Niño and La Niña events may become more intense as the climate continues to warm.1543 Other potential effects, such as shifts in the timing and duration of these events, remain subject to considerable uncertainty.1544
Box 6.1
Major climate influences in the Region
Several broadscale climate drivers interact to influence the Region’s climate, causing it to vary greatly from one year to the next.540,1563,1540 These drivers operate with different spatial patterns and seasonality over timescales from weeks to decades.1540 Some of the most important of these in the Region are the Australian Monsoon, the Madden–Julian Oscillation, the El Niño–Southern Oscillation and the Indian Ocean Dipole.1563,540,1539
The Monsoon is part of the usual evolution of the seasons in northern Australia, with active phases bringing heavy rainfall.1564 It forms when a seasonal reversal of prevailing winds brings a band of low pressure known as the monsoon trough over northern Australia, drawing moist air from the surrounding seas. The monsoon is the major driver of the Region’s rainfall, which occurs mostly in the wet season from December to April.1564 The timing, variability and strength of the monsoon is modulated by a global scale feature of the tropical atmosphere known as the Madden–Julian Oscillation.1565,1566 This eastward moving ‘pulse’ of cloud and rainfall can change the odds of a wetter or drier period on weekly to monthly timescales and increase the chances of cyclones forming in the Region.1565 At the start of the wet season, it can encourage the monsoon trough to form.1565
Over year-to-year timescales, the El Niño–Southern Oscillation is the most important driver of climate variability in the Region and across the tropical oceans.1540 It is also the main contributor to inter-annual cyclical variations in global temperatures.1540 It is associated with a sustained period (many months) of warming (El Niño) or cooling (La Niña) in the central and eastern tropical Pacific Ocean.540 Strong El Niño events cause the centre of Pacific rainfall to move eastward, away from the Australian coast towards South America.1540 Common results include drought across eastern Australia 632 and unusually warm late-summer sea surface temperatures in the region.540,632,633,1567 Strong El Niño events occurred in the Region in 1982–83, 1997–98 and 2015–16, coinciding with worldwide climate extremes.
La Niña years typically bring more clouds, rainfall and cyclones, which can all help to cool the waters over the Reef.1568 The year 2022 marked the third consecutive year of La Niña conditions, a duration reached only 3 times over the past 50 years.582 During extreme La Niña events, the Australian summer monsoon is more vigorous than usual, with more cyclone activity in the Region.538,539 This often results in above‑average rainfall and river flows.
The El Niño–Southern Oscillation interacts with the Madden–Julian Oscillation, and the Indian Ocean Dipole, another major inter-annual climate driver. The Madden–Julian Oscillation can trigger or strengthen El Niño and weaken or disrupt La Niña. The Indian Ocean Dipole can also increase or decrease the intensity of El Niño and La Niña events in the Region.1569,1540 A positive Indian Ocean Dipole may also be associated with warmer-than-average sea temperatures in the Region, and this contributes to the development of marine heatwave conditions.634,246
Projecting climate change involves addressing uncertainties arising from limitations in understanding both the physical climate system and societal trends.1538 The Sixth Assessment Report of the IPCC1538 incorporates both factors using an expanded set of Shared Socio-economic Pathways (SSPs) to model future greenhouse gas concentrations in the atmosphere.1545,1546,1547 These illustrative pathways span the range of possible future emissions scenarios, from very low to very high emissions. For example, SSP1–2.6 portrays a sustainable, low-emissions future resulting in limited warming of 1.8 (very likely range 1.3 to 2.4) degrees Celsius this century; SSP5–8.5 depicts a fossil fuel-driven development path of intensifying emissions resulting in severe global warming in the order of 4 degrees Celsius; while SSP2–4.5 represents a middle-ground scenario in which social, economic and technological trends do not shift markedly from historical patterns.1546
Developments such as emerging climate policy and the rapid growth of renewable energy deployment make very high emissions scenarios like SSP5–8.5 less plausible now than in 2019.1548 Under all considered emissions pathways, the best estimate is for global average temperature to reach 1.5 degrees Celsius above pre-industrial levels between the late 2020s and mid-2030s.1538 The risk of exceeding 2 degrees Celsius depends strongly on emissions pathways, but under medium to high emissions this is likely by the middle of the twenty-first century.1544
Warming of the global climate system is driving climate change. This warming is unequivocal, as confirmed by all major temperature monitoring datasets.608,1549,1550, 1551,1552 It is primarily driven by the release of carbon dioxide and other greenhouse gases due to human activities.1538 In 2023, the carbon dioxide concentration reached a global average of 419.3 parts per million in the atmosphere,1553 the highest levels on Earth in at least 2 million years 540 (Figure 6.4). Globally, 2023 was approximately 1.45 degrees Celsius warmer than pre-industrial levels (with a margin of uncertainty of 1.33 to 1.57) and the past 9 years have been the hottest in the 174-year instrumental record 582,1554 (Figure 6.5).
Figure 6.4
Changes in global atmospheric carbon dioxide concentrations since 1980
Global carbon dioxide concentrations in the atmosphere have been rising for at least the past 173 years, at a faster rate than any time over the past 800,000 years.1541 Global carbon dioxide concentrations (left) rose steadily between 1980 and 2023. The annual mean carbon dioxide rate of increase between 1980 and 2022 (right) shows signs of slowing since 2019. Trends shown are based on globally averaged marine surface data. Source: Lan et al. (2023) 1561

Figure 6.5
Annual global mean surface temperature anomalies (land and ocean)
Global mean surface temperature (GMST) was extracted from the Met Office Hadley Centre for Climate Prediction and Research (HadCRUT5 dataset). Anomalies are with respect to the 1961 to 1990 standard averaging period. The average for 2023 is incomplete with months included to October. The NOAA Oceanic Niño Index (ONI) is defined as the 3-month mean of ERSSTv5 sea surface temperature anomalies in the Niño 3.4 region, based on a rolling 30-year base period. Moderate and strong El Niño and La Niña events in this figure (coloured orange and dark blue respectively) are defined by the ONI being +1.2 °C or above (−1.2 °C or below) in at least one 3-month period during the 12 months from July to June. The asterisks indicate years where notable volcanic activity affected global temperatures. Sources: GMST data from Morice et al. (2021) 1562, ONI data from NWS/NOAA (2023). Adapted from CSIRO and Bureau of Meteorology (2022).540
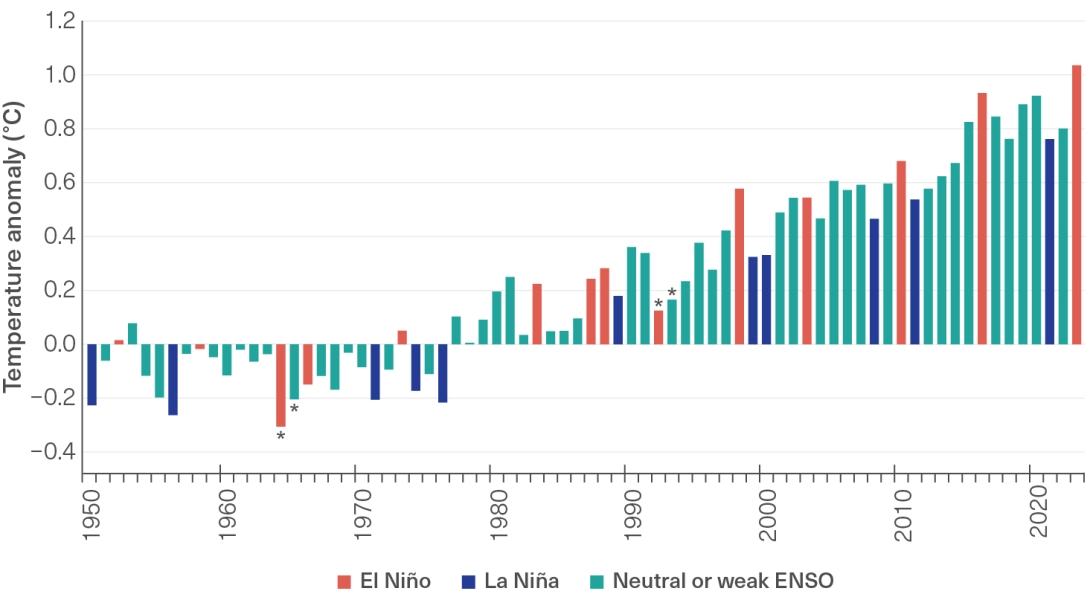
Warming is generally higher over land than over the ocean, but the pace of warming in the oceans is accelerating.1555,540 At the ocean surface, temperature has increased by around 0.9 degrees Celsius since the late nineteenth century, with around two-thirds of this warming occurring since 1980.608 Global surface temperature has increased faster since 1970 than in any other 50-year period over the past 2000 years.1538 The magnitude of these changes exceeds the extent of natural variation over glacial–interglacial time scales.1556,1557,1558 A recent temperature-proxy study on long-lived sponges in the Caribbean indicates that human-caused warming may have begun earlier and may be greater than previously recognised.1559
Around 90 per cent of the extra heat generated by global warming is absorbed by the oceans,1560 causing the heat content of the global ocean to increase since at least 1970.540,582,608 The average warming rate for the surface layers of the ocean (to 2000 metres depth) is 71 per cent higher from 2006 to 2022 compared to a longer baseline (1971 to 2022).582 Ocean heat content is certain to continue to increase over the twenty-first century. Associated warming of the climate system will likely continue until at least 2300, even for low-emissions scenarios, because of the slow circulation of the deep ocean.608 Although continued warming is inevitable, the extent to which the climate will change in coming decades depends on current and future greenhouse gas emissions.1039,1539
The frequency and intensity of marine heatwaves is increasing
The most direct impact of climate change in Australia has been an increase in air and sea surface temperatures.540,1539 Temperatures over Australia have increased by an average of 1.47 degrees Celsius since 1910, at a rate considerably higher than the global average.540 Future warming is likely to be similar to the global average.1539 The number of days with dangerous weather conditions for bushfires has increased in nearly all locations across Queensland 1570 and this trend is projected to continue.540,566 Across the Region, sea surface temperatures are projected to increase by between 0.3 and 1.2 degrees Celsius under a low-emissions pathway by 2030 (relative to a baseline of average temperatures between 1986 and 2005), with little further increase during the twenty-first century.1571,565 However, in a very high emissions pathway, warming would range from 0.6 to 1.3 degrees Celsius by 2030 and 2.3 to 4.7 degrees Celsius by 2090.565,566,1571
Sea temperature increases at the ocean surface in the Australian region have been around 1.05 degrees Celsius since 1900,1572 with the Reef warming by 0.94 degrees Celsius over the same period.660 Since the 1980s, sea surface temperatures have warmed at a rate of between 0.1 and 0.3 degrees Celsius every decade (Figure 3.8). Annual sea surface temperature anomalies for the Region since 1910 are shown in Figure 3.9. Globally, the sea surface temperature is projected to increase on average by about 1.3 degrees Celsius above pre-industrial levels under a low-emissions pathway and by about 3.2 degrees Celsius under a very high emissions pathway.1573,1574 Projected changes to sea surface temperatures across the Region range from 2.1 to 3.5 degrees Celsius by 2090 under very high emissions.565,566,1571 Changes to temperature extremes often lead to greater impacts than changes to the average climate, and these extreme events are becoming both stronger and more frequent because of climate change.582,1538 This trend is projected to continue.1575 A return to near present-day conditions by 2100 is only plausible under a scenario that embraces large net negative emissions to limit warming to 1.5 degrees Celsius.159
Globally, marine heatwaves have doubled in frequency since the 1980s and become longer-lasting, as well as more intense and extensive.608,1572,1576,1577 Despite La Niña conditions (Box 6.1), 58 per cent of the global ocean surface experienced at least one marine heatwave during 2022.582 This trend will continue, with marine heatwaves at the global scale expected to increase four- to eight-fold by the end of this century, depending on emissions pathways.608 In the Reef and Coral Sea, marine heatwaves are increasing in frequency, intensity and extent.246
Globally, average sea levels are rising as a direct consequence of anthropogenic global warming.613 Two main processes are driving sea-level rise: thermal expansion (an increase in the volume of sea water as it warms) and increased melting of land-based ice.540,614
Global average sea level rose faster during the twentieth century than in any prior century during the past 3000 years. Since the late 1960s, sea level rise has accelerated from 3.7 (2006 to 2018)608,615,1578 to 4.6 (2013 to 2022) 582 millimetres per year. Global average sea level reached a new record high for the satellite altimeter record (1993 to 2022) in 2022.582
Increasing loss of land ice from glaciers and ice sheets, including a four-fold increase in the contribution of meltwater from Greenland and Antarctica in 2010 to 2019 compared to 1992 to 1999, has made this the largest contributor to global sea-level rise since 2006.608 Recent research suggests that a continued trend of ice sheet loss from West Antarctica is now inevitable well beyond 2100, irrespective of future emissions pathways.1579 Global average sea level is projected to rise by 0.15 to 0.29 metres by 2050 and by 0.28 to 1.1 metres by 2100. Sea-level rise could be several tenths of a metre higher (and approaching 2 metres under very high emissions) if Greenland and Antarctic ice sheets disintegrate faster than anticipated.608,1580 Projected sea-level rise across the Region is similar to the global average.565,1571
Sea-level rise caused by climate change is superimposed on the normal, frequent fluctuations in sea level associated with tides, storm surges, and ocean waves (swell and wind waves) in the order of metres. Sea-level fluctuations are also affected by cyclical variations of 20 to 30 centimetres over years or decades caused by climate drivers, such as El Niño–Southern Oscillation.608 As these sea level fluctuations occur on a higher baseline, they will lead to increases in the frequency of sea level extremes,608 including storm surges associated with the passage of cyclones. If increases in the Region (Figure 6.6) are similar to the global average, extreme sea level events that previously occurred around once per century will occur about 20 to 30 times as often by 2050 and 160 to 530 times as often by 2100 compared to the recent past.608,629 Sea-level rise is also expected to interact with the complex bathymetry of the Reef to locally increase or decrease tidal ranges such that some coastal areas will be more affected than others.630
Figure 6.6
Projected sea level change within the Region under a future high emissions scenario
Sea level is projected to rise within the Region under representative concentration pathway (RCP) 8.5 over the period 2080 to 2099 relative to the period 1986 to 2005. Projected changes vary spatially across the Region. Source: Zhang et al. (2017)1581
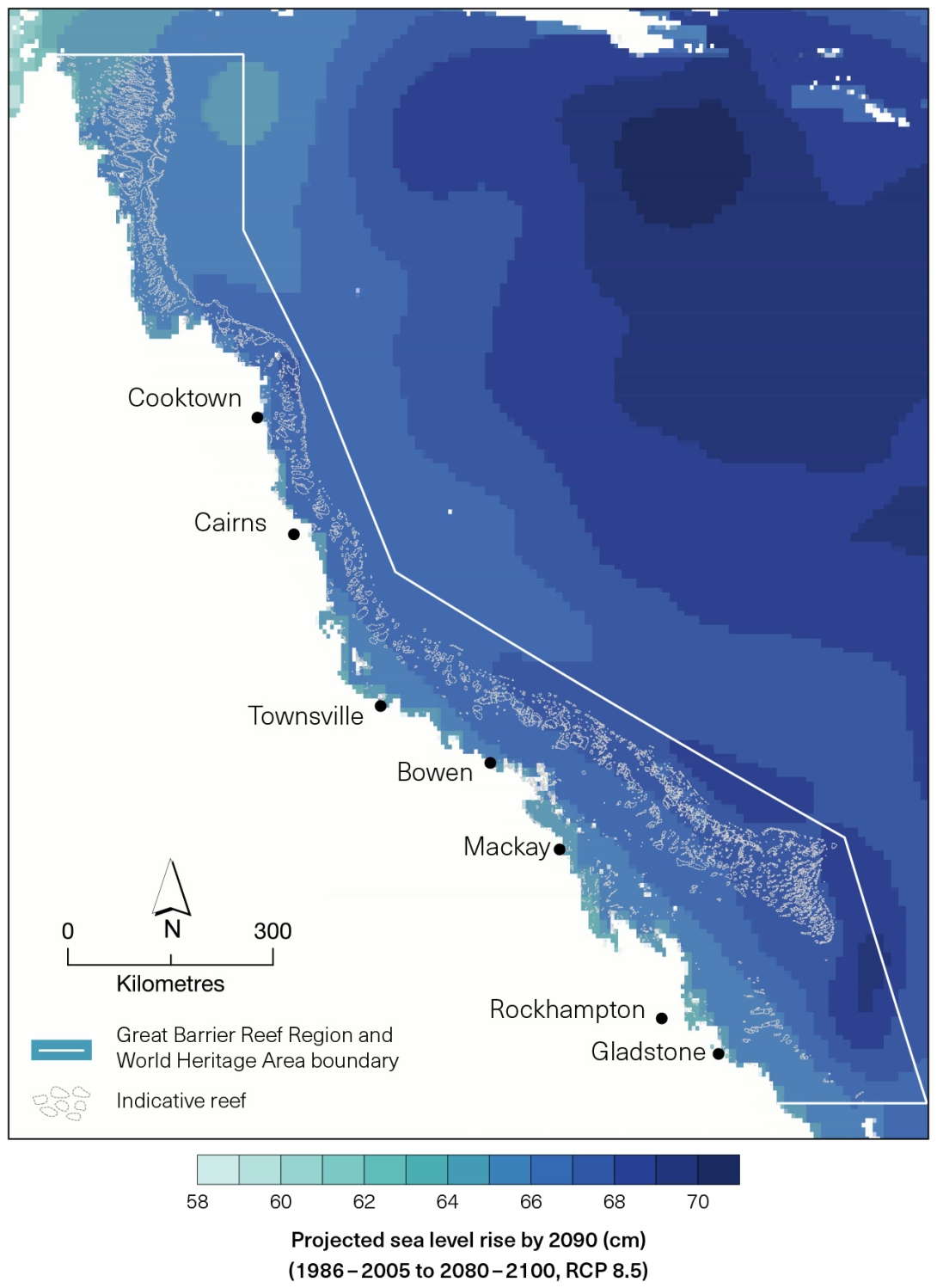
The absorption of carbon dioxide alters ocean chemistry in a process commonly referred to as ocean acidification.1582 The ocean takes up about a quarter of annual global carbon dioxide emissions 1541 and has absorbed roughly one-third of anthropogenic carbon dioxide emissions since the beginning of the Industrial Revolution.1572,1583 The absorption of carbon dioxide leads to a shift in carbonate chemistry involving a decrease in the concentration of carbonate ions and an increase in both bicarbonate and hydrogen ions.1582 This process also lowers the saturation state of aragonite, the form of calcium carbonate used by many marine organisms including reef-building corals, to produce skeletons and shells.1572 One consequence of this process has been a global decrease in ocean pH by approximately 0.1 units (a 26 per cent rise in acidity) since the pre-industrial period (Section 3.3.2)1541,1584,1585 to its lowest level in at least 25,000 years.1541 The increase in acidity is occurring at a rate estimated to be occurring at least 10 times faster than at any time within the past 65 million years.1541,1582,1586 The rapid rate of contemporary changes in ocean chemistry is important in determining the magnitude and impacts of change because the processes by which oceans buffer these changes occur over many thousands of years.1582
Ocean acidification is also progressing rapidly in the Region, with long-term trends predominantly driven by increasing atmospheric carbon dioxide.1539,1587,739 These changes are superimposed on increasing daily and seasonal fluctuations linked to patterns in sea temperatures and stratification, changes in net production and in seasonal transport of offshore waters onto the Reef.535 Concentrations of dissolved carbon dioxide increased by an estimated 6 per cent from 2009 to 2019, and by approximately 28 per cent since 1958.739 The extensively carbonate seafloor has been unable to buffer the seawater against atmospheric changes.739 There are indications that the rate and magnitude of increases of carbon dioxide concentrations are more significant on inshore reefs than those further offshore.1588,1589 For the Reef’s coastal waters, average annual seawater pH is projected to decrease by 2090, compared to that for the period between 1986 and 2005, by an additional 0.15 to 0.33 units under moderate to very high emissions (a 40 to 100 per cent rise in carbon dioxide concentrations, respectively).1571,565 Between 1960 and 2009, the aragonite saturation state likely decreased by 0.33 units.739 Further median declines (compared to 1986 to 2005) of 0.34 to 0.41 units by 2030 and 0.34 to 1.55 units by 2090 are projected to values below critical levels for coral reefs to be able to maintain net calcification, depending on emissions trajectories (Section 6.3.2).535,1571,565
Ocean acidification is directly linked to atmospheric carbon dioxide concentration
Global atmospheric and ocean circulation patterns and rising temperatures influence ocean currents (Section 3.2.1).608 Australian boundary currents and their associated eddies have immediate impacts on the circulation of water over the relatively narrow continental shelf, with flow-on effects for the entire marine ecosystem.1572 The major boundary current affecting the Region, the East Australian Current, has been intensifying in recent decades,1572,608 and this trend is expected to continue.608 This intensification has been associated with an increase in both current strength and the frequency of its southward extensions,1590 contributing to marine heatwaves in south-eastern Australia.1572 More detailed projections of future ocean circulation and altered ocean currents remain a key knowledge gap. The present generation of climate models have a limited ability to forecast the future state of boundary currents, such as the East Australian Current, and do not adequately represent associated eddies or deep ocean and continental shelf interactions.535,1572 Downscaled modelling of climate change projections at resolutions that may better resolve these effects in the Region is underway.535
The number of cyclones has decreased globally during the twentieth century.1591 They have also decreased across northern Australia since reliable satellite observations began in 1982.540 Additional non-satellite observations suggest there has also been a longer-term reduction since 1900.540 The number of cyclones is projected to decrease in the future, but it is expected that a greater proportion will be higher intensity, with continuing large variations in cyclone numbers from year to year (Section 3.2.2).540,1592,1593 Whether or not cyclone-generated waves will become more damaging to coral reefs depends on cyclone size and forward speed as well as intensity. Modelled data are not yet robust enough to make reasonable predictions of these characteristics, especially at fine scales.549 It is projected that average peak wind speeds will increase globally with warming.1575 No climate change effect on spatial extent (size) of cyclones has yet been detected, in part because cyclone size is highly variable, but potential effects are a subject of active research interest.1575 Since 1979, the proportion of intense cyclones has increased by around 25 per cent, which is around 6 per cent per decade and consistent with physical process understanding and climate model predictions.1594,1595 Although observed trends in cyclone intensity remain inconclusive,1596 in part due to the limitations of satellite-based estimates, indirect measurements suggest that cyclones at all intensity categories have strengthened over the past 30 years.542
Model projections indicate that, relative to pre-industrial conditions, cyclones in north-east Australia will have both higher wind speeds (by 5 to 10 per cent on average) and heavier rainfall (by up to 27 per cent for average hourly rainfall rates) under moderate- to high-emissions future climate change.565,1571 Predicted increases in peak rainfall rates have already been substantiated by attribution studies, which indicate that higher temperatures have augmented cyclone-associated rainfall.1596 As a result of this effect, rainfall totals in particular locations are likely to increase in the future, bringing a higher risk of associated flooding.1566 An observed slowdown of forward speed over Australia (by 22 per cent since 1946) suggests flooding risk may also be increased by cyclones remaining over a given location for longer,1597 but future trends in the speed of travel and spatial extent of cyclones remain uncertain.549 In contrast, one of the most assured future impacts from cyclones will be an increase in coastal flooding when storm surges are superimposed on higher sea levels (Section 3.2.5).540,553
Changes in patterns of cyclone activity from year to year may also change over the course of this century.1544,1598 The number of cyclones making landfall in the Region is highly variable over different timescales.537 The frequency of landfall is higher during La Niña years than El Niño years.538,539,1599 This pattern may change; there are indications that cyclones will become more frequent during El Niño events (and less frequent during La Niña events) by the end of the twenty-first century.1544,1598
Observed and projected changes to broadscale rainfall patterns occurring globally 582,1541 and in some regions of Australia 540,1539 are not as evident or well resolved in the Region.565,1571 Recent research has shown that seasonal rainfall has changed in northern Queensland as the climate has warmed, with wet season rainfall increasing and becoming more variable since the pre-industrial period.569 Continued warming of the climate will have important effects on rainfall, particularly through the amplification of extremes of drought and flood. Rainfall in the Region is strongly seasonal, with around 60 to 80 per cent occurring in the wet season from December to April.1564 This rainfall is often associated with the monsoon trough and the passage of low-pressure systems and cyclones, which can bring flooding across the Region (Box 6.1).1564,1600 It is modulated in part by climate drivers, such as the Madden–Julian Oscillation.1565 Variation from year to year is strongly linked to other major climate drivers, especially the El Niño–Southern Oscillation.1565,1601,1602
A warmer atmosphere holds more water vapour
The varied temporal and spatial patterns of rainfall across the Region are superimposed on decadal rainfall trends occurring due to climate change.1539,1541 One of the most important of these is that heavy rainfall events are becoming more intense.540 Daily rainfall totals associated with thunderstorms have increased since 1979, particularly in northern Australia and this trend is expected to continue.540 On a global scale, approximately 20 per cent of global heavy rainfall events over land are attributable to the observed temperature increase since pre-industrial times.1603 For heavy rain days, total rainfall is expected to increase by around 7 per cent for each degree of warming.540,1566 More-intense rainfall increases the likelihood of surface-water and flash floods 1566 (Section 3.2.3) and large flood events are occurring more frequently than in the past (Section 6.5.1).575
Climate across the Region is changing. Changes have already been widely documented in terms of rising air and sea temperatures as well as changing ocean chemistry. Trends for rising sea levels and increasing intensity of storms are increasingly evident. Anticipated changes, such as increases in the intensity of El Niño and La Niña events, vary in certainty but are potentially consequential for the Region’s values.